The genetic testing of race
In one form or another, you may have been (unfortunately) exposed to the notion of ‘testing for someone’s race using genetics.’ In one sense, this is part of the motivation and platform of ‘23andMe’, which maps the genetic variants across the human genome back to likely origin populations to determine the relative ancestry of a person. In a much darker sense, the connection between genetic identity and race is the basis of eugenics, by suggesting genetic “purity” (this concept is utter nonsense, for reference) of a population as justification for some racist hierarchy. Typically, this is associated with Hitler’s Nazism, but more subversive versions of this association still exist in the world: for Australian readers, most notably when the far-right conservative minor party One Nation suggested that people claiming to be Indigenous should be subjected to genetic testing to verify their race.
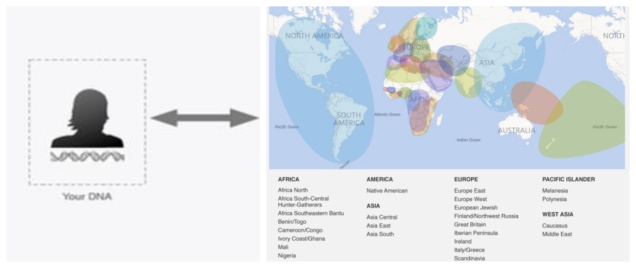
The biological concept of a ‘race’
Beyond the apparent ethical and moral objections to the invasive nature of demanding genetic testing for Indigenous peoples, a crucial question is one of feasibility: even if you decided to genetically test for race, is this possible? It might come as a surprise to non-geneticists that actually, from a genetic perspective, race is not a particularly stable concept.
The notion of races based on genetics has been a highly controversial topic throughout the development of genetic theory and research. Even recently, James Watson (as in of Watson & Crick, who were credited with the discovery of the structure of DNA) was stripped of several titles (including Chancellor Emeritus) following some controversial (and scientifically invalid) comments on the nature of race, genetics and intelligence. Comfortingly, the vast majority of the scientific community opposed his viewpoints on the matter, and in fact it has long been held that a ‘genetic race’ is not a scientifically stable concept.

You might ask: why is that? There are perceivable differences in the various peoples of the world, surely some of those could be related to both a ‘race’ and a ‘genetic identity’, right? Well, the issue is primarily due to the lack of identifiability of genetic variants that can be associated with a race. Decades of research in genetic variation across the global human population indicates that, due to the massive size of the human population and levels of genetic variation, it is functionally impossible to pinpoint down genetic variants that uniquely identify a ‘race’. Human genetic variation is such a beautiful spectrum of alleles that it becomes impossible to reliably determine where one end of the spectrum ends or begins, or to identify a strict number of ‘races’ within the kaleidoscope of the human genome.
How does this relate to 23AndMe?
How does this relate to your ‘23AndMe’ results? Well, chances are that some genetic variants might be able to be traced back to a particular region (e.g. Europe, somewhere). But naturally, there’s a significant number of limitations to this kind of inference; notably, that we don’t have reliable references from ancient history to draw upon very often. This, combined with the fact that humans have mixed among ourselves (and even with other species) for millennia, means that tracing back individual alleles is exceedingly difficult.
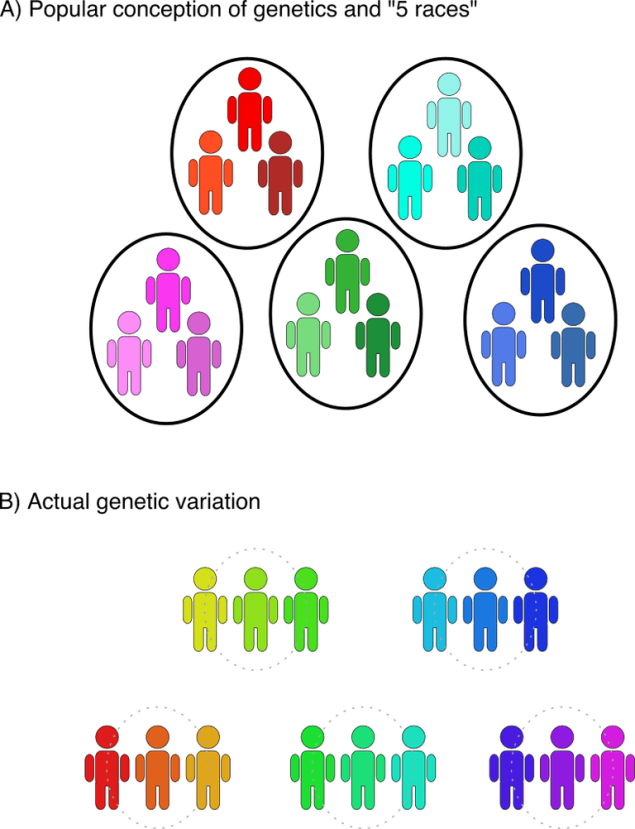
This is exponentially difficult for people who might have fewer sequenced ancestors or relatives; without the reference for genetic variation, it can be even harder to trace their genetic ancestry. Such is the case for Indigenous Australians, for which there is a distinct lack of available genetic data (especially compared to European-descended Australians).
The non-genetic components
The genetic non-identifiability of race is but one aspect which contradicts the rationality of genetic race testing. As we discussed in the previous post on The G-CAT, the connection between genetic underpinning and physicality is not always clear or linear. The role of the environment on both the expression of genetic variation, as well as the general influence of environment on aspects such as behaviour, philosophy, and culture necessitate that more than the genome contributes to a person’s identity. For any given person, how they express and identify themselves is often more strongly associated with their non-genetic traits such as beliefs and culture.
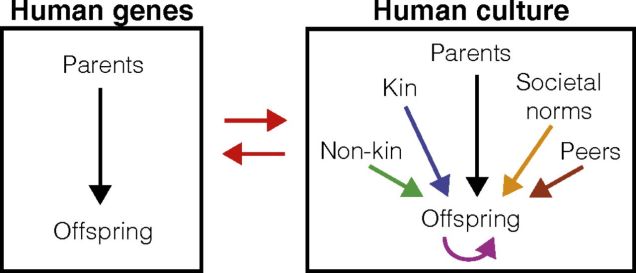
These factors cannot reliably be tested under a genetic framework. While there may be some influence of genes on how a person’s psychology develops, it is unlikely to be able to predict the lifestyle, culture and complete identity of said person. For Indigenous Australians, this has been confounded by the corruption and disruption of their identity through the Stolen Generation. As a result, many Indigenous descendants may not appear (from a genetic point of view) to be purely Indigenous but their identity and culture as an Indigenous person is valid. To suggest that their genetic ancestry more strongly determines their identity than anything else is not only naïve from a scientific perspective, but nothing short of a horrific simplification and degradation of those seeking to reclaim their identity and culture.
The non-identifiability of genetic race
The science of genetics overwhelmingly suggests that there is no fundamental genetic underpinning of ‘race’ that can be reliably used. Furthermore, the impact of non-genetic factors on determining the more important aspects of personal identity, such as culture, tradition and beliefs, demonstrates that attempts to delineate people into subcategories by genetic identity is an unreliable method. Instead, genetic research and biological history fully acknowledges and embraces the diversity of the global human population. As it stands, the phrase ‘human race’ might be the most biologically-sound classification of people: we are all the same.