Science in pop culture
For most people, scientific research can seem somewhat distant and detached from the average person (and society generally). However, the distillation of scientific ideas into various forms of media has been done for ages, and is particularly prevalent (although not limited to) within science fiction. It’s not all that uncommon for scientists to describe the origination of their scientific interest to have come from classic sci-fi movies, tv shows, or games. I’m not saying dinosaurs haven’t always been cool, but after seeing them animated and ferocious in Jurassic Park, I have no doubt a new generation of palaeontologists were inspired to enter the field. I’m sure the same must also be at least partially true for archaeology and Indiana Jones. While I can guarantee the actual scientific research is nowhere near as adventurous and high-octane thriller as those movies would depict, their respective popularities renew interest in the science and inspire new students of the disciplines.

The inclusion of science within pop culture media such as movies, tv shows, music and video games can have profound impacts on the overall perception of science. This influence seems to go either way depending on how the science is presented and perceived: positive outlooks on science can succinctly present scientific matter in a way that is easy to interpret, and thus can generate interest in the fields of science. Contrastingly, negative outlooks on science, or misinterpretations of science, can drastically impact what people understand about scientific theory. For example, despite being a horrendously outdated belief, Lucy proposed that the average human only uses 10% of their brain capacity: achieving 100% brain capacity using a stimulant, the titular character becomes miraculously superhuman. While this concept is clearly outrageously behind the times for anyone who follows psychological sciences, a disturbing number of people apparently still believe this notion. Thus, misrepresentation of scientific theory perpetuates outdated concepts.

Don’t get me wrong: I love ridiculous science fiction as much as the next nerd, and I’m certainly not of the expectation that all science-based information needs to be 100% accurate, without fail (after all, the fiction and fantasy has to fit somewhere…). But it’s important to make sure the transition from scientific research to popular media doesn’t lose the important facts along the way.
Evolution’s relationship with pop culture has been a little more complicated than other scientific theories. Sometimes it’s invoked rather loosely to explain supernatural alien monsters (e.g. Xenomorphs; Alien franchise); other times it’s flipped on its head to show a type of de-evolution (Planet of the Apes). Science fiction has long recognised the innovative and seemingly endless possibilities of evolution and the formation of new species. Generally, the audience is fairly familiar with the concept of evolution (at least in principle) and it makes for a useful tool for explaining the myriad of life in science fiction stories.
Evolution in video games?
It probably doesn’t come as a huge surprise to note that I’m a nerd in all aspects of my life, not just my career. For me, this is particularly a love of video games. Rarely, however, do these two forms of nerdism coincide for me: while some games apply science and scientific theory, they are usually biased towards physics and engineering disciplines (looking at you, Portal). As far as my field is concerned, there are a few notable examples (such as Spore) which encapsulate the essence and majesty of evolution, but rarely do they incorporate the ‘genetic’ aspect that I love.

You can then imagine my utter delight at the discovery of a game that actually incorporates both population genetics and interesting gameplay. The indie survival game, aptly named Niche: A Genetics Survival Game, very literally represents this ‘niche’ for me (and I will not apologise for the pun!). Combining simplified models of population genetics processes such as genetic diversity, inbreeding (and associated inbreeding depression), natural selection, and stochastic events, Niche beautifully incorporates scientific theory (albeit toned down to a layman level) with challenging, yet engaging, gameplay mechanics and adorable art style.
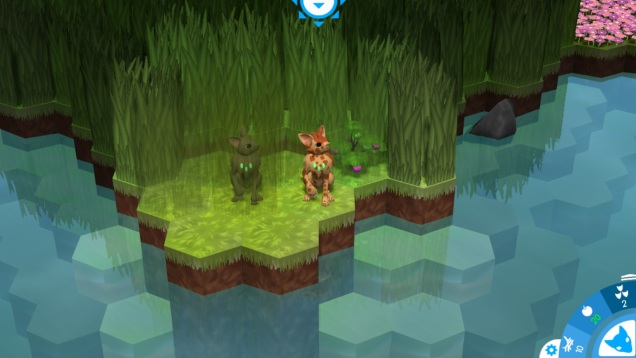
As one might expect from the title, Niche is at heart a survival game: the aim is to have your very own population of animals (dubbed ‘Nichelings’) survive the stresses of the world, through balancing population size, gene pools, resources (such as food, nests, space) and fighting off predators. Over time, the genetics component drives the evolution of your Nichelings, pushing them to be better at certain tasks depending on the traits selected for: the ultimate aim of the game is to create the perfectly adapted species that can colonise all of the land masses randomly generated.

Niche requires cunning strategy, good foresight and planning, and sometimes a little luck. Although I’m decidedly not very good at Niche yet (I think my rates of extinction would mirror the real world a little too much for my liking…), the chance to involve my scientific background into my favourite hobby is a somewhat magical experience.

You might wonder why I care so much about a video game. While the game is in and of itself an interesting concept, to me it exemplifies one way we can make science an enjoyable and digestible concept for non-scientists. It’s possible that Niche could open the door of population-level genetics and evolution to a new audience, and potentially inspire the next generation of scientists in the field. Although that might be an extraordinarily long shot, it is my hope that the curiosity, mystery and creativity of scientific research is at least partially represented in media such as gaming to help integrate science and society.
Using video games for science?!
Both science and society can benefit from the (accurate) representation of science in pop culture, not just through fostering a connection between scientific theory and the recreational hobbies of people. In rare occasions, pop culture can even be used as a surrogate medium for testing scientific theories and hypotheses in a specific environment: for example, World of Warcraft has unwittingly contributed to scientific progress. As part of a particular boss battle, characters could become infected with a particular disease (called “Corrupted Blood”), which would have significant effects on players but only for a few seconds. While this was supposed to be removed after leaving the area of the fight, a bug in the game caused it to stay on animal pets that were afflicted, and thus become a viral phenomenon when it started to spread into the wider world (of Warcraft). The presence of the epidemic wiped out swathes of lower level players and caused significant social repercussions in the World of Warcraft community as players adjusted their behaviour to avoid or prevent transmission of the deadly disease.
This unique circumstance allowed a group of scientists to use it as a simulation of a real viral outbreak, as the spread of the disease was directly related to the social behaviour and interactivity of players within the game. The “Corrupted Blood” incident such enthralled scientists that multiple papers were published discussing the feasibility of using virtual gaming worlds to simulate human reactions to epidemic outbreaks and viral transmissions on an unparalleled scale. Similarities between the method of transmission and behavioural responses to real-world events such as the avian flu epidemic were made.

This isn’t the only example of even World of Warcraft informing research, with others using it to model economic theories through a free market auction system. While these may seem extraordinarily strange (to scientists and non-scientists alike), these examples demonstrate how popular media such as gaming can be an important interactive front between science and society.